Halobacterium cells can use both oxygen and light as energy sources (1). The most efficient source of energy for Halobacteria is oxygen. However, light can also be used as an energy source because bacteriorhodopsin, a protein found in the membrane of Halobacteria, allows phototropic energy production (2). Although oxygen is the most efficient source of energy for Halobacteria, the presence of oxygen does not prevent Halobacteria from using some light as an energy source. Therefore, in the presence of oxygen and light, bacteriorhodopsin is produced.
Bacteriorhodopsin is a protein with numerous potential applications in the fields of biotechnology and bioengineering, because it functions as a light driven proton pump (3). When the bacteriorhodopsin captures a photon of light, the energy causes an isomerization change of the retinal molecule (bound to the center of the bacteriorhodopsin), which then causes bacteriorhodopsin to undergo a series of conformational changes. During the photo-cycle of bacteriorhodopsin, various amino acids are protonated and deprotonated. The net result of the photo-cycle is that a proton passes through the protein membrane, moving from the cytoplasmic fluid to the extracellular fluid (4).
Bacteriorhodopsin is a trans-membrane protein found in the cellular membrane of Halobacterium salinarium (5). The bacteriorhodopsin is composed of seven alpha helices that arrange in a homotrimer within a hexagonal 2-D crystalline array in the membrane of H. salinarium. A molecule of retinal chromophore (a derivative of Vitamin A) is bound to Lys216 by a Schiff-base (4). The membrane of H. salinarium is composed of lipids and bacteriorhodopsin, which are present in a proportion of three to one respectively. |
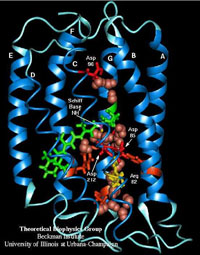 |
Recently various researchers have developed two different hypotheses (though not necessarily discrete) to explain the primary determinant of the directionality of the proton transfer (6). In other words, these researchers are trying to rationalize the observed movement of the proton. The “accessibility switch” hypothesis postulates that the directionality of the proton transfer occurs because conformational changes undergone by the bacteriorhodopsin structure during the photo-cycle switch the access of various key groups on the pathway from the extracellular to the cytoplasmic side (7). Based on this model, the Schiff-base can be accessed extracellularly early in the photo-cycle, but after conformational changes Schiff-base can only be accessed from the cytoplasm. Therefore, deprotonation and re-protonation of the Schiff-base is affected by accessibility.
In contrast, the “affinity switch” hypothesis attributes the directionality of the proton transfer to the relative proton affinities of various key groups along the pathway. The impetus for the proton’s directionality comes from the affinity changes that occur along the photo-cycle allowing various groups to act as proton donors or proton accepters (8).
Researchers at ISB are working to develop a bacteriorhodopsin lab for high school students, so students can learn about the conditions that affect production of the purple membrane. The lab in development compares the production of bacteriorhodopsin under aerobic conditions with and without light to the production of bacteriorhodopsin under anaerobic conditions with and without light. The bacteriorhodopsin production can be measured quantitatively by using a spectrophotometer, as well as qualitatively by observing the color change of the samples. When Halobacterium culture grows in the presence of oxygen and light, the bacteriorhodopsin produced causes the culture to become pinky-lavender in color. When Halobacterium grows anaerobically in the presence of light, although bacteriorhodopsin is produced, the sample becomes salmon orange in color after 48 hours, and no purple can be detected from the bacteriorhodopsin growth. (--MD)
References
- DasSarma, S.; DasSarma, P. Encyclopedia of Life Sciences. Wiley Press; 2006. Halophiles.
- Müller JA, DasSarma S. Genomic analysis of anaerobic respiration in the archaeon Halobacterium sp. strain NRC-1: dimethyl sulfoxide and trimethylamine N-oxide as terminal electron acceptors. J Bacteriol. 2005;187:1659–1667.
- Lanyi, J. K. Bacteriorhodopsin as a model for proton pumps. Nature. 1995;375:461—463.
- Onufriev, A., Smondyrev, A. & Bashford, D. Proton affinity changes driving unidirectional proton transport in the bacteriorhodopsin photocycle. J. Mol. Biol. 2003;332:1183-1193.
- DasSarma, S., Berquist, R., Coker, J., Dassarma, P. & Müller, J. Post-genomics of the model haloarchaeon Halobacterium sp. NRC-1. Saline Systems. 2006;2.
- Lanyi, J. K. Bacteriorhodopsin. Curr. Opin. Struct. Biol. 11, 415-419.
- Oesterhelt D, Stoeckenius W. Rhodopsin-like protein from the purple membrane of Halobacterium halobium. Nat New Biol. 1971;233:149–152.
- Brown, L. S., Diomaev, A. K., Needlman, R. & Lanyi, J. K. Cennectivity of the retinal Schiff base to Asp-85 and Asp-96 durting the bacteriorhodopsin photocycle: the local acess model. Biophys. J. 75, 1455-1465.
|